Case Studies
B S C RAO
CASE STUDIES - Learning Through Failure
Failures occur - that is a fact. Forensic engineering goes behind the scenes and discovers the why, what, when and how of what happened to cause the failure, and while doing so also discovers who were the principal actors in the story and whether, and to what extent, they were responsible. In addition, it also highlights all the contributory faults from which we could learn many useful lessons to improve our understanding of human, structural and material behaviour. From these follow recommendations that would prevent such errors from happening this making the practice of civil and structural more precise, safer and more reliable. Failures thus become great teachers. History is replete with examples of how structural failures have been instrumental in bringing about profound changes in design and construction. There have also been almost-failures, which were detected in time and prevented by the courageous and ethical conduct of the person(s) involved which are inspiring for all. Here we look at some examples.
The Tacoma Narrow Bridge - "Galloping Gertie"37,46,47,48
The famous suspension bridge was supposed to have been the third-longest bridge in the world when it was completed (1940), with a total length of 5,939 feet (1,811m), comprising a 2,800 ft. (854m) centre span and two 1,100 ft. (335m) end spans. It was supposed to have withstood winds up to 120 miles per hour (192kmph), but would flutter under the smallest breeze, and failed violently at just 42 mph (67kmph) on 7th November 1940. Why, when the art and science of suspension bridge design was already over a hundred years old by then? Failures of suspension bridges during windstorms were well known in the 19th century. Seven bridges in the US and three in Europe had either collapsed or suffered severe damage during windstorms, so that they were considered dangerous and unreliable.46 However, during that time when railways dominated transportation in the US, the failure of these bridges was attributed to the passage of heavy trains and overloading rather than to the effects of wind. The effects of wind forces were also considered and provided for in their design, but only by making the bridges stiffer to resist horizontal wind load, using latticed stiffening girders. The crucial significance of latticed girders vis-a-vis the wind was not understood. Longer and longer bridges were being successfully built, and changes in technology were taking place.37 The earliest suspension bridges had used ropes, which were replaced with linked iron bars and chains (Menai Strait Bridge, 1826) and soon thereafter wrought iron wire cables. In 1883, steel wire cables were introduced in the building of the Brooklyn Bridge designed by John Roebling, then America’s most famous suspension bridge designer.46
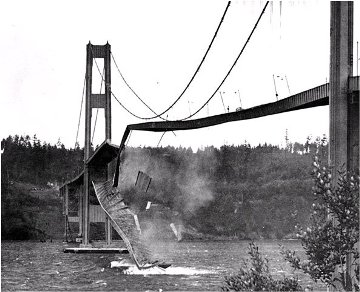 |
Fig. 20 Collapse of the Bridge
Fig. 22 Eldridges Design with Lattice Girder48
In 1909, Leon Moisseiff used the "deflection theory" to design the Manhattan Bridge. However, the "deflection theory" had been developed in Austria for the design of concrete arch bridges, which Moissief further developed for suspension bridges. This theory held that stiffening trusses and cable-stays were unnecessary and that just the dead weight of the bridge alone was enough to withstand the lateral effects of wind pressure (It was then assumed that wind only applied lateral pressure; the vertical motion effects of wind were not understood). Just stiffen the bridge to take the additional lateral wind stresses and the bridge could be light and strong was the ruling philosophy. The effect of this theory on bridge design was dramatic, because it could reduce the weight of bridges by several hundreds of tons, the cost by several millions of dollars and looked much sleeker and more graceful, the last being most appreciated.46
In 1935 the George Washington Bridge became the longest suspension bridge in the world with a centre span of 3,500 feet (1,067m), and in 1937 the famous Golden Gate Bridge with an even longer span of 4,200 feet (1,280m). Both of these used a lattice-truss deck structure. In 1939, the Bronx-Whitestone Bridge introduced a radical departure from conventional design by using solid plate girders instead of trusses to support the deck, and using rigid frame towers with no diagonal cross bracing.46 The confidence of bridge designers was surging with the successful completion of these massive projects. And then the Tacoma Narrows Bridge was built with the same plate-girder design and minimal stiffening and opened to traffic on July 1, 1940. Four months later, on November 7, 1940 the bridge oscillated violently in a 42 mph (67 kmph) wind and was literally torn apart and collapsed into Puget Sound. Remarkably no one was killed, but for a lone dog which had to be abandoned in a stranded car on the bridge. The whole failure was captured on 16mm movie film by a local photographer and has some dramatic footage (http://www.youtube.com/watch?v=j-zczJXSxnw).
So what happened? It may be best explained by what Diane Vaughn, while commenting on the Challenger Space Shuttle explosion, described as "normalisation of deviance" ie. "the gradual acceptance of sequential minor errors and failures, accumulating and culminating in a major catastrophe". The series of successful bridges in the previous years had imbued designers with such supreme confidence (over-confidence?) in their knowledge and abilities, that they brushed aside contrary evidence.
Fig. 21 Self Induced Oscillation48
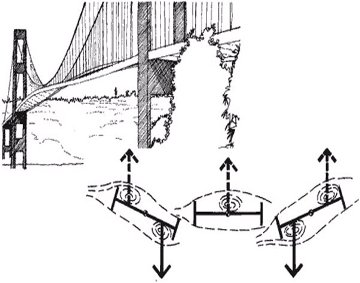 |
Bridge users had observed the tendency of these new light, long and slender bridges to oscillate on windy days, but that was explained away by bridge designers as a minor trade off against the aesthetics, lightness and economy of the bridges.37 The Tacoma Narrows Bridge was, at the time, the most slender suspension in the bridge in the world. The designers also "forgot" the suspension bridge design lessons of the previous century and amplitude failed to account for the dynamic effects of wind. The solid plate girders supporting the bridge deck acted as barriers to wind flow below the deck, while the wind flowed smoothly above, causing an aerodynamic uplift. Soon the bridge started oscillating and grew in until finally the deck structure ruptured and the bridge collapsed. It has always been popularly, but incorrectly, been explained as a classic case of "harmonic resonance" (or forced oscillations),but the more accurate explanation is now held to be a case of "aero-elastic torsional flutter" (self-induced oscillation) (Fig.21).47 However, even seven decades later the debate still rages on as to what exactly caused the failure, and the jury is still out.48
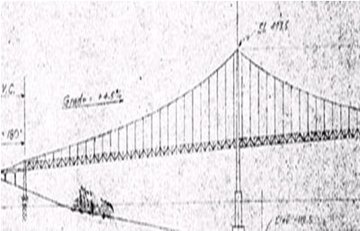 |
Fig. 22 Eldridges Design with Lattice Girder48
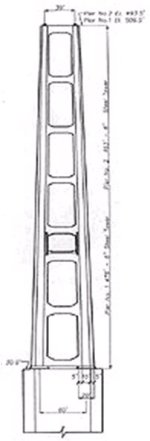
Fig. 23 Eldridge's Tower48
|
But this might not have happened at all. A bridge across the Tacoma Narrows had been long felt, but finance was a problem. Clark Eldridge, a Washington State engineer, produced a tried-and-tested conventional bridge design, with a centre span of 2,600 feet (793m) and end spans of 1,300 feet (396m) each, using a 25-foot (7.62m) deep lattice-truss deck structure (Fig. 22) and braced towers [463ft 6in (141m) and 476ft 6in (145m) high], with five bracing struts above the deck and three below (Fig.23).46However, when the Washington Toll Bridge Authority requested the Federal Public Works Administration (PWA) for USD 11 million to build the bridge, Leon Moissief wrote to the PWA offering to build the bridge at much lower cost. His design, he said, would be much slimmer, much lighter and more elegant, and would cost USD 4 million less.49 The PWA approved his proposal, and Moissief completely revised the design of bridge, with a central span of 2,800 feet (854m) and end spans of 1,100 feet (335m), plate girder deck structure 8 ft. (2.44m) deep and with a reduced tower height (both 425 ft, 129.5m) having only two struts each above and below the deck. However, owing to some problems at the site Eldridge’s foundation design was retained. Moissief’s bridge had a span-to-width ratio of 72, significantly greater than 47 for the Golden Gate Bridge. It was the slenderest and lightest bridge (for its span) at the time - and that ultimately proved to be its undoing. But, would Eldridge's bridge have survived the conditions on that fateful day of 7th November 1940? Modern opinion is Yes. But would it have survived the design wind speed of 120mph? No one has given an answer.48
|
This leaves one last question for us to mull over. Was Leon Moissief right in what he did? Was he guilty of professional negligence? Remember - he was one of the leading and most respected suspension bridge designers of his day. In actual fact though, he was not found guilty of negligence because, as per the contemporary wisdom in the design of suspension bridges prevailing at that time which held that these new bridges were quite different from those of the previous century, he had acted correctly.37
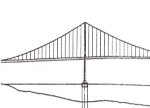
|
The aftermath of this structural disaster was a tremendous surge of research and development in new mathematical theories of vibration, wave phenomena, aerodynamics and harmonics, suspension bridge design and construction and mandatory use of wind tunnel testing which have subsequently governed the design of all of the world’s long span bridges.
Fig. 24 Moissief’s Design with Plate Girder48
|
Epilogue: The wreckage of the destroyed first Tacoma Narrows Bridge still remains in the waters of Puget Sound, as a reminder of the imperfect understanding of man and the great power of wind. The Second Tacoma River Bridge was subsequently built in 1950, designed with much greater understanding. From the lessons learned from the Tacoma disaster, the Bronx-Whitestone Bridge, which also used a plate-girder supported deck, was later extensively retrofitted to withstand the design wind forces.
Engineer's Liability - The Case of the Unreinforced Masonry Wall37
New building regulations required that an unreinforced masonry wall built around 1888 had to be strengthened to resist seismic loading. The structural engineering of record (SER) had produced the designs and drawings, and the design drawings and specifications required the contractor to submit the shoring and bracing plans to the SER for approval. But the contractor proceeded with construction without those plans and without the knowledge of the SER. Both the owner and the contractor excluded the SER from the construction activities and no construction plans were submitted to him for review and approval. They did not also carry out the required shoring and bracing - perhaps to save money. This resulted in the brick wall collapsing and killing the foreman on duty. But the SER was sued for negligence and wrongful death.
Two years later, and after a six-week trial, the jury acquitted the SER and found him not negligent nor liable, but instead found both the owner, and through the indemnity clause in the construction contract, the contractor guilty by wilfully ignoring the specifications and preventing the SER from discharging his responsibility, and awarded damages of over USD 1 million to the family of the deceased foreman.
A few important issues are to be noted here. This "simple" and apparently "straightforward" accident was not what it appeared to be, and it required two years of investigations to unravel the real truth behind what had happened. Very often - and this is something that we in India constantly experience - clients and owners force engineers into designing and building structures at the lowest cost (the infamous "L1" bid that public sector organisations are so fond of accepting) and in the shortest time leading to dangerous and very risky short cuts being adopted. The risk in such situations is great and the consequences terrible.
The Abbeystead Disaster - Methane Gas Explosion50,51,52
Abbeystead is a small picturesque village in Lancashire, in the northwest of England. The area was subject to periodic flooding during winter. It was also the location for an (unmanned) underground water pumping station, which was built to pump up to 62 million Imp. Gallons (280,000 cu.m) of water every day from the River Lune, about 12 km away, near Lancaster into the River Wyre, near Abbeystead. From here water was again pumped, through a buried pipe and concrete tunnel, into a water treatment plant to supply drinking water to South Lancashire. Locals in the area believe that the pumping of water from the River Lune to the River Wyre was the cause of the flooding.
Fig.25 Schematic of the Water Supply Scheme at Abbeystead
The Setting The North West Water Authority (NWWA), which was in charge of the whole scheme, wanted to allay the fears of the residents and so invited a group of them from the nearby village of St. Michael's, about 16 km away from the pumphouse, on the river Wyre for a demonstration at the pump house to show that pumping was not the problem. On May 23, 1984, a group of 44 people visited gathered at the pumping station to witness the demonstration. There being no cause for any anxiety the visitors were allowed to smoke within the pump house. Owing to conditions of drought at the time there had been no pumping for about 16 days previously and the water level had fallen low in the tunnel, and a void had formed above the water.
The Explosion The pumps were then switched on and immediately there was a blinding flash and a massive explosion. It destroyed the pump house and blew up the roof, lifting some 30 beams each weighing about 2.5 tons into the air through the soil landscaping above, before they crashed down again, instantly killing eight of the visitors and seriously injuring the rest. Eight more died of their injuries later. The dead included two children. (Fig.26)
Fig. 26 After the Explosion (New Scientist 31-05-1984)
Why? A court of inquiry was ordered, and the investigators found traces of methane in the pump house and tunnel. The pipeline/tunnel were buried in the earth and there were coal deposits about 1000 metres below ground level. The immediate cause of the explosion was considered to be that methane, percolating from the coal deposit had been accumulating in the empty pipeline. When the pumps were switched on, the water rushing through the pipe had forced the methane into the pump house through the set of valves and a spark of some kind (the exact trigger was never established)set off the massive explosion. A second version that the author remembers reading in a (now-forgotten) journal was that methane had been accumulating in the closed unmanned pump house over the years, and when the pumps were switched the gas exploded instantly.
The Investigation - In the aftermath of the accident/disaster, there was a detailed inquiry which lasted for three years. Compensation claims totalling £4 million50 were preferred against the consulting engineers (Binnie and Partners), the contractor (Edmund Nuttall Ltd.) and the operator (NWWA) for negligence. The consultants were held responsible for not having conducted sufficient investigations into, and not anticipating, the possibility/probability of methane entering the system, considering that it was entirely underground and there were known coal deposits (albeit at 1000m below GL). It was contended that had they done so, they would have been able to detect the presence of methane and provide adequate ventilation to prevent a build-up of the gas. The contractors were charged with being negligent in not testing for methane during construction, and the operator was accused of not having foreseen the possibility of methane and testing for the same after the tunnel was built and before starting the pumps on that unfortunate day.
All three claimed that they were not responsible and denied liability. The consultant said he had carried out adequate tests and had not found any methane and that the accumulation of methane could not have been reasonably foreseen. Both the consultant and contractor also argued that there was no reason to check methane during construction as it would have escaped into the atmosphere, even if it had been percolating from the coal deposits below. In any case they argued that contractually their obligations and responsibilities for the system ended in December 1980. The operator said that there was no reason for him to suspect the accumulation of methane since they had been successfully operating the pipeline and pumps for five years prior to the accident, since June 1979 when the system was commissioned, without any problems.51
It is worthwhile pointing out here that methane is a very dangerous and highly explosive gas when its concentration exceeds 5% by volume, and it was suspected that the concentration in the pump house had reached between 5 and 15%.
The Verdict - The court case went on for three years and in March 1987, the Lancaster High Court found that the consultant, Binnie& Partners, was liable for 55% in negligence for failing to exercise "reasonable care" in assessing the risk of methane. The contractor, Edmund Nuttall Ltd, was liable for 15% for not conducting systematic tests for methane, and the operator, NWWA, liable for 30% for not ensuring that pump house was safe for the visitors and employees. All three appealed, but finally only Binnie and Partners were found entirely responsible! Their appeal to the House of Lords was refused.52As a result Binnie and Partners went into bankruptcy and closed down.
The Health and Safety Executive investigated the accident and prepared a special report as required by the Health and Safety at Work Act 1974.
Case 2: The 59-Storey Crisis - Citicorp Centre - A Case of Ethical Conduct53,54,55
Wiliam LeMessurier (1926-2007) (pronounced "le measure") was an eminent structural engineer in the US who was responsible for the design of many famous buildings in the USA and abroad including the Federal Reserve Bank of Boston and the Singapore Treasury Building. He also built the landmark Citicorp Headquarters Building(Fig.27) in New York city (Citicorp Centre) with its striking 45o wedge-shaped roof. With a height of 915 feet (279m) and fifty-one stories, it is now the53 eighth tallest building in New York City, and was the seventh tallest in the world when it was completed in 1977. It was, and still is, a unique building for another important reason too.
Fig. 27 The Citicorp Building
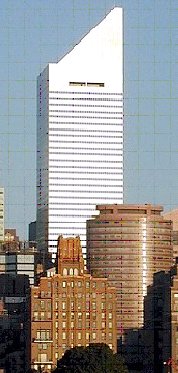 |
When Citigroup wanted to build its new headquarters on Lexington Avenue, it chose a site which was directly across the street from its then headquarters. However, the site was occupied on the northwest corner by a crumbling St. Peter’s Church, built in 1905, but Citigroup wanted the whole site. So the church was able to negotiate a very advantageous deal : Citigroup could occupy the whole site - but above the church - provided that Citigroup also built a new church in the same place where it now stood! The church traded the air rights above it for a new church, but created a major problem for the bank, since the main columns could now not be at the corners of the site.
LeMessurier was the structural engineer and came up with an innovative idea of having four massive columns 114 feet (35m) high on the centre of each side instead of at the corners, with the bank building projecting (cantilevered) 72 feet (22m)over and above the church. It was a radical and very innovative solution - and very brave, but it was a magnificent "eye-catcher" unlike anything seen before, and the huge skyscraper "seemed to float" above the street ! It was a magnificent achievement. The building was built and opened in 1977.
|
About a year later, in June 1978, Le Messurier got a phone call from an engineering student who said he had been given an assignment to write a report on the Citicorp Centre, and wanted to clarify some points. When the two met the student told him that he had been taught that tall buildings must have their main columns at the corners and not at the sides; so how is it that this building had columns on the sides. Le Messurier was nice to the young man and promised to call him back later, which he did explaining that it was necessitated by the site conditions.
Fig. 28 The Citicorp Building Plaz
But the question set him thinking - and that made all the difference. What he found, after some deep thought, (to cut a long, but fascinating, story short) was that, while there was nothing wrong with the concept, or the design, of the building with its columns at the centre of each of the four sides, there was indeed a serious flaw in the design of the building! He found that "quartering winds" (ie. those blowing diagonally across the building) increased the strain in some areas by more than 40%.53He had also discovered, to his horror a few weeks earlier, that the welded joints he had designed had been replaced with bolted joints (albeit with the approval of his firm) and further that the number of bolts provided (four) were half of what was required (eight), since only perpendicular winds, and not the worse-case diagonal winds, had been considered.
Very worried, he had a wind-tunnel test done, and it confirmed his worst fears. He found that the building would topple over in a 16-year storm and not the 100-year design storm, ie. there was a 1 in 16 chance that the building would collapse within a year.53 Compounding the problem was the building’s weight. At 25,000 tonnes it was unusually light compared with similar tall buildings like the Empire State Building which weighed in at 60,000 tons. However, to reduce sway, a 410-ton tuned mass damper had been installed on the upper stories of the tower54but that required electricity, which could fail during storm conditions.
He immediately informed Citicorp of his findings, and an emergency plan was put into operation including evacuation plans in the event of a storm, and getting weather forecasts three times every day. Strengthening works were started in August, with addition of 2-inch thick steel plates and additional welding in all of the more than 200+ critical bolted joints of the building. Welders worked through the night and carpenters and other trades during the day round the clock for three months till October. Interestingly, the public was never aware of all this drama. Midway during the repairs, Hurricane Ella appeared off the coast of New York and seemed to be heading for Manhattan, but luckily it turned towards the sea and the tower escaped what might have been a calamity. After all the repairs were completed the building was re-evaluated and found to be one of the sturdiest buildings, and able to withstand a 700-year storm.52
The cost of the repairs was about $ 8 million, but Le Messurier's insurance could only provide $ 2 million. After much negotiation with Citibank's lawyers, he was able to persuade them to accept the $ 2 million settlement.
It is a tribute and a testimony to the extraordinary honesty, courage and integrity of William Le Messurier that he blew the whistle on himself, took responsibility and paid for it. That’s ethical conduct of the highest order!
"You have a social obligation. In return for getting a license and being regarded with respect, you're supposed to be self-sacrificing and look beyond the interests of yourself and your client to society as a whole. And the most wonderful part of my story is that when I did it nothing bad happened." Le Messurier
The entire story was revealed only twenty years later, in 1995, in a detailed and brilliant article in the New Yorker magazine by William Morgenstern entitled "The 59-Story Crisis".56
ASSOCIATIONS AND INSTITUTIONS FOR FORENSIC ENGINEERING
Overseas, forensic engineering is a robust, well-recognised and represented and thriving subject with many professional institutions and practitioners, consultants and courses in academic institutions. Some of these are mentioned below for information.
-
National Academy of Forensic Engineers, New York
nafe@nafe.org
-
International Board Of Forensic Engineering Sciences, Florida, USA
http://www.iifes.org/
-
Engineering Sciences Section of the American Academy of Forensic Sciences
-
The Society of Forensic Engineers and Scientists, California
http://www.forensic-society.org/
-
Technical Council on Forensic Engineering, ASCE
http://www.asce.org/Content.aspx?id=2147488650
-
American Board of Forensic Engineering and Technology
http://www.abfet.us/
-
The College of Investigative and Remedial Consulting Engineers of Australia, CIRCEA,
http://www.circea.org/
-
The Association of Consulting Forensic Engineers (ACFE), Dublin Ireland
http://www.forensicengineers.ie/
Indian Scenario
In India, unfortunately, while forensic science is well-known, forensic engineering is virtually unknown. There is no association or institution either at the local or national level to neither represent forensic engineers or forensic engineering, nor do any academic institutions teach the subject as a separate discipline. In fact, even the subject of forensic engineering is hardly known to engineers in this country. Perhaps, therefore, the time has come, as India grows in stature and as a global power, to create a professional Institution of (or Indian Society of) Forensic Engineers to give this special field of engineering expertise greater exposure and strength.
Conclusion and Acknowledgements
This article has been an attempt to provide broad overview of the whole and exciting field of forensic (civil) engineering and illustrate, with a little detail, the various facets of this very interesting discipline, which is relatively unfamiliar to Indian civil engineers. It is a vast field of knowledge and obviously it is not possible, in other than a treatise, to cover all aspects in any great detail or depth. Readers may be disappointed that some issues have been omitted or not covered in sufficient detail.
There is nothing of any original contribution by me in this article, which is a compilation and consolidation of information from many diverse sources, which I have only re-presented in my own fashion. My research to write this article has been a highly educative and rewarding journey and I a much the richer for it. I hope that you too may find something of use. It would not have been possible without the extensive literature available in the public domain and accessible through the Internet, and I humbly acknowledge the great debt of gratitude I owe to the authors of all these literature. I have therefore tried, as rigorously as possible, to quote the references to the statements made but, again, it is possible that I may have inadvertently missed some. I have also given the URLs for all my sources which I have accessed on the Internet. For all my errors and omissions I take full responsibility and offer my humble apology, and will be grateful if they are pointed out to me by readers.
References Cited In Four Cover Feature Articles by Er. BSC Rao
- Fig.1, 2,3
- Wikipedia - Hyatt Regency Walkway Collapse Fig.4 ;
- ( Source: Fig.5)
- Wikispace - Kansas City Hyatt Regency Walkways,
- Hyatt Regency Walkways Collapse - Engineering Ethics - Texas A&M University, This Presentation Guide provides step-by-step instruction for a debate concerning the building failure. This paper discusses ethical and structural issues surrounding the building failure.
- Wikipedia Forensic engineering;21052012
- Carper, Kenneth. L, 2001; What is Forensic Engineering, CRC Press LLC
- Noon, Randall K, 2001; “Forensic Engineering Investigation”, CRC Press
- Greenspan, H F, O’Kon, J A, Beasley, K J and Ward, J S (1989) Guidelines for Failure Investigation, ASCE:New York
- http://www.worldscibooks.com/engineering/6335.html
- Sanders, Jan, Dipl.Inf., 2012, Introduction to Why Because Analysis, http://www.rvs.uni-bielefeld.de/research/WBA/
- Wikipedia - Falsifiability, http://en.wikipedia.org/wiki/Falsifiability
- Gelder,Pieter van et.al (April 2008); Reliability Analysis of Flood Sea Defence Structures, Report No. T07-08-01; FLOODSite Consortium, European Commission
- The Open University - Introduction to Forensic Engineering
- US Department of Energy (1999), Conducting Accident Investigations Work Book – Revision 2, Excellent resource
- Sklet, Snorre (2002) Methods of Accident Investigation, NTNU Norwegian University of Science and Technology, Reliability, Safety and Security Studies; http://www.ntnu.no/ross/reports/accident.pdf
- Bulsuk, Karn G (April 2009); An Introduction to 5-Why
- Bulsuk, Karn G (April 2009); 5-WhyWeaknesses,
- Wikipedia - Why-Because Analysis,
- Wikipedia - fault Tree Analysis,
- Fault Tree Analysis, Weibull - Reliasoft Corporation, Ericson II, Clifton A(1999);
- Fault Tree Analysis, Fault Tree Analysis
- Logic Gates, Power Point Presentation, http://www.edutek.ltd.uk/Binaries/GCSE/LogicGates.ppt
- Vesely, W E et al (January 1981), Fault Tree Handbook, NUREG-0492, Systems and Reliability Research, Office of Nuclear Regulatory Research, US Nuclear Regulatory Commission,Washington DC 20555 A
- Clemens, P.L (May 1993);Fault Tree Analysis - 4th edition
- The Development of Wind Damage Bands for Buildings, Journal of Wind Engineering and Industrial Aerodynamics,Vol.84, Issue 1, January 2000
- Rausand, M (October 2005), Chapter 3 System Analysis Failure Modes, Effects, and Criticality Analysis, Department of Quality Engineering,Norwegian Technological University
- Understanding Risk Priority Numbers, Ombu Enterprises,LLC
- Risk Priority Number (from Failure Modes and Effects Analysis) (2012), Institute of Healthcare Improvement, Cambridge, Massachusetts
- Analyze Opportunity Part 1. Failure Modes Effect Analysis; Purdue University
- Weibull 1992, Basic Concepts of FMEA and FMECA Reliasoft Corporation
- Expert Testimony the free dictionary
- Parakh, S C (2011), Expert Witness, Medico Legal Issues,2011, Vol. 55 No.4 pp 421-22
- The Indian Evidence(Amendment) Bill, 2003,
- Black, Justice M E J, (May 2008); A Practice Direction: Guidelines for Expert Witnesses in Proceedings in the , Federal Court of Australia or Federal Court of Australia
- Daley, Judge Timothy The Lectric Law Library © 1995-2012
- Kardon, Joshua B (March 1999); The Structural Engineer's Standard of Care, Online Ethics Center for Engineering National Academy of Engineering
- Srinivasan, V, Halada, Gary and Quinn, Jim (August 2008), Engineering Disasters and Learning from Failure, Department of Materials Science and Engineering, State University of New York at Stony Brook
- Matousek,M and Schneider, J., (1976), Untersuchungen Zur Struktur des Zicherheitproblems bei Bauwerken, Institut für Baustatik und Konstruktion der ETH Zürich, Bericht No. 59, ETH
- Krishnamurthy, Dr. Natarajan (Nov. 2007), Forensic Engineering in Structural Design and Construction, Structural Engineering World Congress 2007
- Schmidt, Jon A (Jan 2009), The Definition of Structural Engineering, Structure Magazine, January 2009 issue
- BAJI (Bench Approved Jury Instruction) 6.37, "Duty of a Professional." California Jury Instructions, Civil. West Publishing Company, January, 1986.
- Responsibility, Accountability, and Liability: Studies in the Theory of Responsibility for Engineering Ethics and Engineering Accountability, Drexel University
- American Society of Civil Engineers, Code of Ethics
- Institution of Engineers (India), Code of Ethics for Corporate Members
- Washington State Department of Transportation, Tacoma Narrows Bridge: 1940 Narrows Bridge, The Machine
- Billah, Yusuf and Scanlan, Bob (1991), Resonance, Tacoma Narrows bridge failure, and undergraduate physics textbooks, Am. J. Phys. 59 (2) February 1991
- Washington State Department of Transportation, Tacoma Narrows Bridge: Lessons From the Failure of a Great Machine
- Wikipedia, Tacoma Narrows Bridge (1940)
- Wikipedia, Abbeystead
- Eckersley & Others v. Binnie & Others
- Wikipedia, Abbeystead Disaster
- William LeMessurier and the CitiCorp Building
- When Design is a Matter of Life or Death
- Allan Bellows, A Potentially Disastrous Design Error
- Joe Morgenstern (May 1995), "The Fifty-Nine Story Crisis", The New Yorker, May 29,1995, pp 45-53
References Used But Not Cited In Article
- Risk Analysis Methodologies,
- Journal of Performance of Constructed Facilities Volume 25, Issue 6 (December 2011)
- Das, S. and Chew, M. (2011), Generic Method of Grading Building Defects Using FMECA to Improve Maintainability Decisions, Journal of Performance of Constructed Facilities, 25(6), 522–533.
- Performing Failure Mode and Effect Analysis
- Gregory, Frank Hutson (1992) Cause, Effect, Efficiency & Soft Systems Models , Warwick Business School Research Paper No. 42 (ISSN 0265-5976), later published in Journal of the Operational Research Society, vol. 44 (4), pp 333-344
- Wikipedia, Engineering Ethics,
- Galloping Gertie
- Today's Tacoma Narrows Bridge
- Comparison of the Bridges
- Tacoma Narrows Bridge
- Ethics in Engineering, State University of New York at Stony Brook.Excellent List of Codes of Professional Ethics
- A great website on Galloping Gertie