Application of bacteria
As self-healing agent for sustainable concrete
Henk M. Jonkers, Arjan Thijssen, Gerard Muyzer, Oguzhan Copuroglu, Erik Schlangen
Introduction
Concrete is a strong and relatively cheap construction material and is therefore presently the most used construction material worldwide. One drawback, however, is that its massive production exerts negative effects on the environment. Its main ingredients, i.e. cement and aggregate material, need to be produced and mined on massive scale and transported over considerable distances increasing energy consumption, greenhouse gas emissions and landscape mutilation. It is estimated that cement (Portland clinker) production alone contributes 7% to global anthropogenic CO2 emissions, what is particularly due to the sintering of limestone and clay at a temperature of 1500 degree Celsius, as during this process calcium carbonate (CaCO3) is converted to calcium oxide (CaO) while releasing CO2 (Worrell et al., 2001). Therefore, from an environmental viewpoint, concrete does not appear to be a sustainable material (Gerilla et al., 2007). Another aspect of concrete is its liability to cracking, a phenomenon that hampers the material's structural integrity and durability. The impact of durability-related problems on national economies can be substantial and is reflected by the sums of money spent on maintenance and repair of concrete structures. It is estimated that in the United States alone the annual direct cost for maintenance and repair of concrete highway bridges due to reinforcement corrosion amounts to 4 billion dollars (FHWA, 2001).
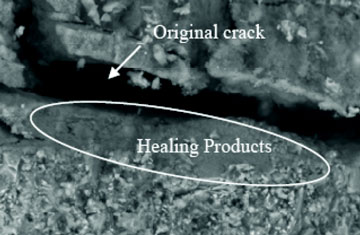 |
Particularly cracking of the surface layer of concrete reduces material durability as ingress water and detrimental chemicals cause a range of matrix degradation processes as well as corrosion of the embedded steel reinforcement (Neville, 1996). In order to reduce the production costs, several industrial by-products such as fly ash, silica fume and blast furnace slag are nowadays commonly used as clinker (cement) replacements in concrete mixtures. Besides reducing the costs this practice also contributes to a more sustainable material as significant amounts of clinker can be saved, e.g. in the Netherlands in cement type CEM III/B more than 65% of clinker is replaced by blast furnace slag. Although for decades the need to produce more sustainable cement-based products is thus recognized and implemented, the development of sustainable maintenance and repair methods for concrete constructions is still in its infancy. Durability problems such as crack formation are typically tackled by manual inspection and repair, i.e. by impregnation of cracks with cement or epoxy-based or other synthetic fillers (Neville, 1996).However, a promising sustainable repair methodology is currently being investigated and developed in several laboratories, i.e. a technique based on the application of mineral-producing bacteria.
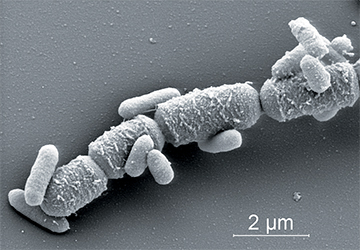 |
The application of bacteria for ecological engineering purposes is becoming increasingly popular as is reflected by recent studies where bacteria were applied for removal of chemicals from waste water streams (Gross et al., 2007), for bioremediation of contaminated soils (Chaturvedi et al., 2006) and removal of greenhouse gasses from landfills (Jugnia et al., 2008). The applicability of specifically mineral-producing bacteria for sand consolidation and limestone monument repair (Gollapudi et al., 1995; Stocks-Fischer et al., 1999; Bachmeier et al., 2002; Dick et al., 2006; Rodriguez- Navarro et al., 2003) and filling of pores and cracks in concrete have been recently investigated (Bang et al., 2001; Ramachandran et al., 2001; De Muynck et al., 2008a,b; Ramakrishnan, 2007). In most of these studies ureolytic bacteria of the genus Bacillus were used as agent for the biological production of calcium carbonate based minerals. The mechanism of calcium carbonate formation by these bacteria is based on the enzymatic hydrolysis of urea to ammonia and carbon dioxide. As the pK-value of the equilibrium constant is close to 9.2 the reaction causes a pH increase from neutral to alkaline conditions resulting in the formation of bicarbonate and carbonate ions which precipitate with present calcium ions to form calcium carbonate minerals. A potential drawback of this reaction mechanism is that for each carbonate ion two ammonium ions are simultaneously produced which may result in excessive environmental nitrogen loading. On the other hand, the method proved promising for repair of cracks in concrete as regained strength (Bang et al., 2001; Ramachandran et al., 2001; Ramakrishnan, 2007) and decrease of material permeability (De Muynck et al., 2008a,b) was observed. In all studies so far, bacteria or derived ureolytic enzymes were externally applied on cracked concrete structures or test specimens, i.e. as surface treatment or repair system. Besides the aforementioned potential environmental drawbacks of the urease-based system, manual inspection and external application of bacteria on cracked areas of concrete structures is labor intensive as it is repeatedly needed during the service life of a construction and is therefore expensive.
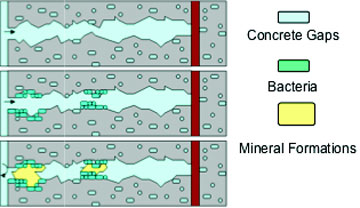 |
In order to avoid these drawbacks, the main goal of this research project was to test the applicability of alternative metabolic mineral-producing pathways by bacteria which are truly incorporated in the concrete matrix. Such bacteria added to the concrete mixture prior to casting should remain viable for prolonged periods once integrated in the concrete matrix and be able to produce copious amounts of minerals needed to plug or seal freshly formed cracks. Integrated bacteria would thus represent an internal self-healing agent which autonomously decreases matrix permeability upon crack formation. An integrated healing agent would save manual inspection and repair and moreover increase structure durability. Addition of such an agent to the concrete mixture would thus save both money and the environment as less maintenance and use of environmental unfriendly repair material is needed. Main challenge of this research project was therefore to identify suitable bacteria which not only survive concrete incorporation for prolonged periods, but also can additionally act as self-healing agent.
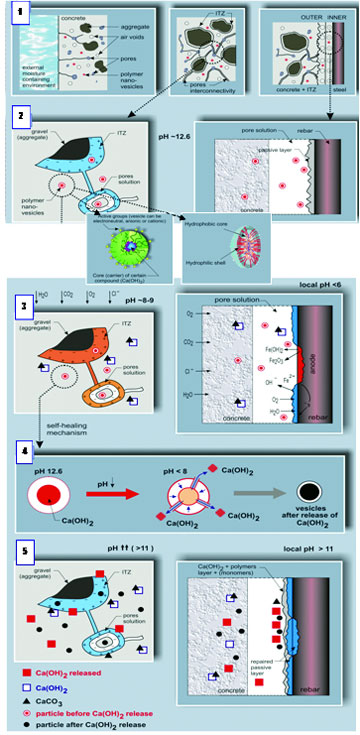 |
Methods
Selection and cultivation of bacteria
The matrix of fresh concrete is highly alkaline particularly due to the formation of portlandite (calcium hydroxide) which is after calcium-silica-hydrate quantitatively the most important hydration product of ordinary Portland cement (OPC). Matrix capillary water of young concrete is typically characterized by pH values between 11 and 13. Bacteria added to the concrete mixture thus do not only have to resist mechanical stresses due to mixing but should also be able to withstand a high alkalinity for prolonged periods. Most promising bacterial agents for incorporation in the concrete matrix therefore appear to be alkaliphilic (alkali-resistant) spore-forming bacteria. As the concrete matrix is oxic due to ingress oxygen (diffusion through matrix capillaries) incorporated bacteria also need
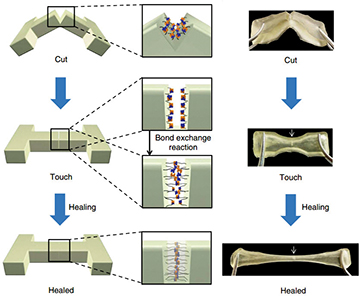 |
to be oxygen tolerant. Such aerobic alkaliphilic spore-forming bacteria occur within the genus Bacillus, and several representatives of these were therefore selected to test their applicability as healing agent in concrete. Two species originally isolated from alkaline soil samples, Bacillus pseudofirmus DSM 8715 and B. cohnii DSM 6307, were purchased from the German Collection of Microorganisms and Cell Cultures (DSMZ), Braunschweig, Germany. These bacteria were cultured in liquid media according to the suppliers' recommendations (medium composition: 5 g peptone, 3 g meat extract, 0.42 g NaHCO3 and 0.53 g Na2CO3 per liter distilledwater;pH9.7) and also in alkaline mineral medium supplemented with manganese to enhance sporulation (spore formation), what was rather poor in peptone/meat extract medium. The alkaline medium contained per liter of MilliQ ultra-pure water 0.2 g NH4Cl, 0.02 g KH2PO4, 0.225 g CaCl2, 0.2 g KCl, 0.2 g MgCl2·6H2O, 0.01 g MnSO4·2H2O, 1ml trace element solution SL12B, 0.1 g yeast extract, 5.16 g citric acid trisodium salt, 4.2 g NaHCO3 and 5.3 g Na2CO3. The pH of this medium was close to 10. Cultures were aerobically incubated in Erlenmeyer flasks on a shaker table at 150 rpm. Growth and sporulation yield of bacteria was regularly checked and quantified by microscopic analysis. Senescent cultures containing high number of spores were washed by repeated centrifugation and re-suspension of the cell pellet in sterile tap water to harvest vegetative cells and spores but to remove dissolved culture constituents and residues. Obtained suspensions were microscopically analyzed to quantify the number of present bacterial spores, and the suspension was subsequently kept in a fridge at 4 ?C until further use as cement paste admixture (see below).
Preparation of cement stone test specimens
Cement stone specimens with and without incorporated bacteria (a mixture of vegetative cells and bacterial spores) were prepared to investigate viability of incorporated bacteria, pore size distribution of aging specimens, effect of agent additions on strength and self-healing properties. Ordinary Portland cement (CEM I 32.5R, ENCI, The Netherlands) was mixed with aliquots of tap water in a water-to-cement weight ratio of 0.4 or 0.5. Bacteria containing specimens were prepared by addition of washed spore suspensions replacing part of the makeup water. Liquid paste was poured in moulds (with dimensions of 4 cm×4cm×4 cm), gastight sealed and cured at room temperature. The curing time of test specimens depended on the type of experiment done (see below). Bacterial specimens contained 1-10×108 sporescm−3 cement stone.
Viability test of cement stone incorporated bacteria
The survival rate of incorporated bacterial spores (2.4×108 B. cohnii spores cm-3 cement stone; specimens (4cm×4cm×4cm) prepared with a water-to-cement weight ratio of 0.5) in time was determined by estimation of the number of viable bacteria present in aged (9, 22, 42 and 153 days cured) cement stone specimens. This was done by application of a standard microbiological dilution-to-extinction method, i.e. the most-probable-number (MPN) technique. In short, aged cement stone specimens were crushed to powder in a hand-held mortar, suspended in nine aliquots of medium, homogenized by repeated mixing on a vortex mixer and ultrasonic treatment in a water bath, and subsequently serially diluted (eight replicates) to extinction in eleven consecutive 10-fold dilution steps in alkaline growth medium in 96-wellsmicrotiter plates. Growth in positive wells could visually easily be recognized by increased turbidity of the medium. MPN numbers (estimate of viable cells present in the original sample) were calculated with a computer program according to Clarke and Owens (1983).
Strength and pore size distribution of aging cement stone specimens
The development of pore diameter size distribution and potential deleterious effect of agent additions on compressive strength development in aged (3, 7 and 28 days cured) cement stone specimens (4cm×4cm×4 cm) was investigated. Pore size distribution of specimens prepared with a water-to-cement weight ratio of 0.5 was determined by mercury intrusion porosimetry (MIP) using a Micromeritics AutoPore IV Mercury Porosimeter. Aged cement stone specimens were cut to smaller pieces with a chisel (fragment sizes about 0.5cm×0.5cm×0.5 cm) which were frozen in liquid nitrogen and subjected to cryo-vacuum evaporation for at least 2 weeks for pore water removal prior to MIP testing. Compressive strength development of similarly aged cement stone specimens (4cm×4cm×4 cm) prepared with awater-to-cement weight ratio of 0.4 and with either incorporated bacterial spores (6×108 B. pseudofirmus sporescm-3) or some organic compounds (yeast extract, peptone, calcium acetate and calcium lactate) from which bacteria can potentially produce bio-minerals, was determined using a manual compressive strength pressure bench. Organic compounds were added to the cement paste mixture in a quantity of 1% of cement weight.
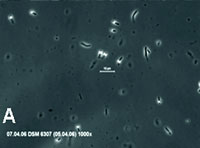
|
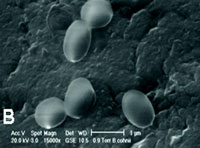
|
Fig. 1. Optical photomicrograph (1000× magnification) of B. cohnii culture showing vegetative cells with intracellular spores (bright spheres) (A) and ESEM photomicrograph (15,000× magnification) of isolated B. cohnii spores, showing that spore diameter sizes are up to 1µm (B). |
Mineral-producing capacity of healing agent-incorporated specimens
Cement stone specimens (4cm×4cm×4 cm) without (control) and with incorporated bacterial spores (1×108 cm-3 B. cohnii spores) plus calcium lactate (0.5% of cement weight) prepared with a water-to-cement weight ratio of 0.5were broken to pieces after 7 or 28 days curing. Pieces were subsequently incubated in tap water at room temperature for 8 days where after mineral formation at crack surfaceswas determined by environmental scanning electron microscopy (ESEM) using a Philips XL30 Series ESEM. Specimen pieces were rinsed in distilled water and subjected to ESEM analysis without any further sample treatment.
Results
Bacterial spore formation
Addition of manganese to the growth medium stimulated the formation of bacterial spores substantially. Light microscopic analysis of growing cultures (Fig.1A) revealed that sporeswere produced within vegetative cells (endospores). Single spores remained in culture after senescence of vegetative cells. Spores could be easily visualized by ESEM analysis due to their thick cell walls (Fig. 1B), and their diameter appeared to be typically in the size range of 0.8-1µm.
3.2. Viability of cement stone incorporated spores
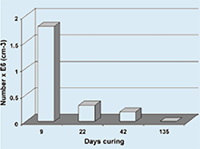 |
Application of the MPN technique allowed estimation of the number of viable bacterial cells (vegetative cells plus bacterial spores) present in aging cement stone specimens. For the MPN procedure bacteria had to be freed from the cement stone matrix and ideally brought in a single cell (non-clumped) suspension.
As for this procedure cement stone had to be crushed and pulverized using high mechanical force and suspended by ultrasonic treatment, a relatively low yield could be expected. From the 9 days cured specimens about 1%, i.e. 1.8x106 of the incorporated 2.4x108 sporescm-3 cement stone were retrieved (Fig. 2), a value that is not extraordinary low considering the previous arguments.
|
Fig 2. Most-probable-number estimate of viable bacterial spores (B. cohnii) incorporated in aged cement stone specimens
|
However, as the number of viable cells substantially decreased with increasing specimen age it could be concluded that viability of cement stone incorporated spores decreased in time. The number of viable cells from a 135 days cured cement stone specimens was below the MPN detection limit (<0.5x103 cm-3) indicating that the viability of the majority of spores which were directly mixed with the cement paste mixture was below 4 months. Control cement stone specimens which were prepared from cement paste without purposely added bacteria and tested simultaneously did not yield viable bacteria. This indicates that the non-sterile tap water that was used as makeup water contained, if any, less than 0.5×103 cm−3 cement stone-resistant bacteria.
Pore diameter size distribution and strength of cement stone specimens
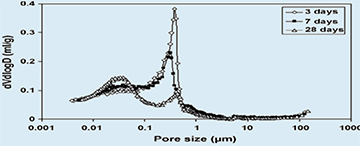
|
Fig. 3. Pore diameter size distribution of aged cement stone specimens (without bacteria) |
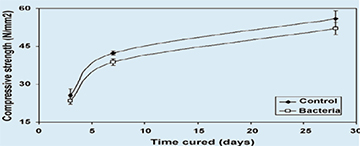
|
Fig. 4. Development of compressive strength of aged control and bacteria incorporated (B. pseudofirmus) cement stone specimens. |
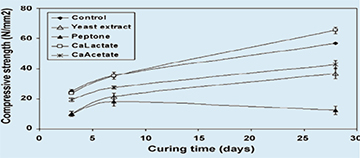
|
Fig. 5. Development of compressive strength of aged control and organic compound-incorporated cement stone specimens |
The distribution of pore sizes as well as strength development of aging cement stone specimens with or without incorporated agents was determined. MIP analysis revealed two major cement stone matrix pore diameter size classes (Fig.3), i.e. of 0.01-0.1 and 0.1-1µm. A clear shift in relative abundance from larger pores in young specimens (3 and 7 days cured) to smaller pores in older (28 days cured) specimens is apparent. The trend towards smaller pores shows a fast decrease in 0.8-1µm-sized pores, the typical size class of bacterial spores, in aging cement stone samples. Compressive strength tests showed that addition of different potential healing agents to the cement paste mixture had widely varying effects on aging specimens. Incorporation of a high number of bacterial spores (6×108 cm−3) resulted in a decrease in strength of less than 10% for 3, 7 and 28 days cured specimens (Fig. 4). The majority of added organic compounds, i.e. three out of four, also resulted in a significantly reduced strength development (Fig. 5). While calcium acetate and yeast extract additions lowered compressive strength values to about half of that of the control, the addition of peptone appeared completely detrimental. Only the incorporation of calcium lactate did not substantially affect strength (3 and 7 days cured) or even resulted in a slight increase (28 days cured) in compressive strength values.
Mineral production capacity of test specimens
Control cement stone specimens (no bacteria included) cured for 7 or 28 days produced small, typically 1-5µm-sized particles on crack surfaces (Fig. 6). A prominent feature on crack surfaces of young specimens (7 days cured) is massive production of fibrous material not visible on crack surfaces of older (28 days cured) specimens. The crack surface of healing agent-containing cement stone specimens (bacteria plus calcium lactate) appeared to be different. Copious amounts of larger 20-80µm-sized mineral-like particles were formed at the crack surface of 7 days but not on those of 28 days cured specimens (Fig. 7). In fact, crack surfaces of older, 28 days cured, control and healing agent-containing specimens appeared not substantially different (Figs. 6C and D and 7C and D).
Discussion
The main objective of this study was to establish whether bacteria incorporated in the cement stone matrix could act as self-healing agent to catalyze the process of autonomous repair of freshly formed cracks. One major problem associated with crack formation is that the process results in a drastic increase in material permeability increasing the risk of matrix and embedded reinforcement degradation by ingress water and other aggressive chemicals. Active bacterially mediated mineral precipitation could result in crack-plugging and concomitant decrease in material permeability. As bacteria function as catalyst, a suitable mineral precursor compound needs additionally to be incorporated in the material matrix to provide a truly autonomous repair mechanism. However, the maximal allowable amount of mineral precursor compound introduced to the concrete mixture is likely limited as larger quantities may negatively affect other concrete properties such as setting time and (final) strength. Therefore, the addition of smaller quantities of mineral precursor compounds will probably result in autonomous repair of micro- (<1mm diameter) but not in healing of larger (macro) cracks. Here we have shown that bacteria plus calcium lactate, representing a two-component healing agent, mediate the production of copious amounts of 20-80[1]m-sized mineral-like precipitates on crack surfaces of young, 7 days cured, cement stone specimens. Although the effectiveness of decreasing material permeability still needs to be established, the process appears promising particularly because ESEM analysis indicates that copious and robust minerals are produced (Fig. 7A and B).
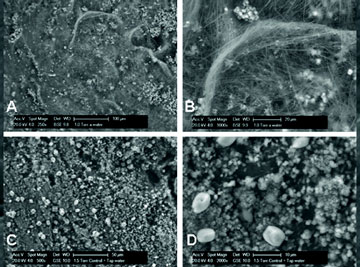
|
Fig. 6. Cement stone specimens without incorporated healing agent (controls), cracked after 7 (panels A: 250× and B: 1000× magnification) or 28 days curing (panels C:500× and D: 2000× magnification). |
The minerals are likely calcium carbonate-based and form due to bacterial metabolic conversion of calcium lactate according to the following reaction:
CaC6H10O6 +6O2→ CaCO3 +5CO2 +5H2O
The yield of calcium carbonate-based minerals will even increase when produced CO2 molecules react with portlandite (Ca (OH)2) minerals, which are quantitatively important hydration products
of cement particles, according to:
5CO2 +Ca (OH) 2→ 5CaCO3 +5H2O
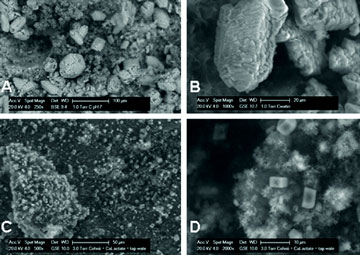
|
Fig. 7. Cement stone specimens with incorporated healing agent (B. cohnii spores plus calcium lactate), cracked after 7 (panels A: 250× and B: 1000× magnification) or 28 days curing (panels C: 500× and D: 2000× magnification). The relatively large (20-80µm sized) mineral precipitates visible on crack surfaces of young specimens (A and B) are presumably due to bacterial conversion of calcium lactate to calcium carbonate. The small (2-5µm sized) precipitates on crack surfaces of older specimens (C and D) resemble those produced by abiotic specimens (see Fig. 6C and D), larger bacterial precipitates are not produced here likely due to loss of viability of cement stone embedded bacterial spores. |
The latter reaction is homologous to carbonation, a slow process that naturally occurs in concrete due to inward diffusion of atmospheric carbon dioxide, which rate is however substantially enhanced by the metabolic conversion of calcium lactate.
The process of bacterial mineral formation from calcium lactate represents an alternative mechanism to the urease-based system investigated in previous studies. In contrast to the latter mechanism, metabolic conversion of calcium lactate does not result in production of massive amounts of ammonia what drastically increases the risk of reinforcement corrosion (Neville, 1996) and degradation of the concrete matrix particularly when further oxidized by bacteria to yield nitric acid (Diercks et al., 1991). However, a striking phenomenon in this study is that the functionality of bacterial mineral production of the incorporated two-component healing agent appears limited to young concrete. Massive production of larger-sized precipitates was observed in 7 days but not in 28 days cured cement stone specimens. The suitability of the bacteria applied in this study as self-healing agent clearly relates to their capacity to form spores, which are known to be able to withstand high mechanical forces and are characterized by a long term viability of up to 200 years under dry conditions (Schlegel, 1993). However, it is observed in this study that apparently time related decreased functionality is likely related to the observed loss of viability of cement stone incorporated bacterial spores (Fig. 2). In turn, the loss of spore viability appears to be linked to the continuing decrease in matrix pore diameter sizes (Fig. 3) as MIP analysis revealed that the larger size class (0.1-1[1]m pores) still present in young (3 and 7 days cured) specimens almost disappeared in favor of the smaller (0.01-0.1[1]m) size class in 28 days cured specimens. While pores of the larger size class present in young specimens can still accommodate bacterial spores with typical diameters of 0.8-1[1]m (Fig. 1B), the majority of incorporated spores apparently become crushed in aged specimens, resulting not only in loss of viability but also in decreased mineral-forming capacity. A possible solution to the problem of loss of viability and related functionality of incorporated bacterial spores could be provided by encapsulation or immobilization of spores in a protective matrix prior to addition to the concrete mixture. A possibility could be embedding of bacterial spores prior to concrete mixture addition in sol-gel-like materials, i.e. inorganic oxide matrices, as in a previous study it was demonstrated that Bacillus sphaericus spores immobilized in silica sol-gels retained germination ability (Soltmann et al., 2003).
Another possible strategy to avoid crushing of bacterial spores in aging concrete could be the addition of air-entraining agents to the concrete mixture to create isolated micropores in the concrete matrix in which spores can survive. In conclusion we can state that alkali-resistant spore-forming bacteria related to the genus Bacillus represent promising candidates for application as self-healing agent in concrete and probably other cement-based materials. We found evidence that cement stone incorporated bacterial spores are able to convert concomitantly incorporated calcium lactate to calcium carbonate-based minerals upon activation by crack ingress water. Our continuing research focuses on obtaining a substantially increased time related functionality as well as on the quantification of the self-healing capacity and thus increased durability of bacteria based self-healing concrete.
Acknowledgement
Financial support from the Delft Centre for Materials (DCM at: www.dcmat.tudelft.nl) for this work is gratefully acknowledged.
References
- Bachmeier, K.L., Williams, A.E.,Warmington, J.R., Bang, S.S., 2002. Urease activity in microbiologically-induced calcite precipitation. J. Biotechnol. 93, 171-181.
- Bang, S.S., Galinat, J.K., Ramakrishnan, V., 2001. Calcite precipitation induced by polyurethane-immobilized Bacillus pasteurii. Enzyme Microb. Technol. 28, 404-409.
- Chaturvedi, S., Chandra, R., Rai, V., 2006. Isolation and characterization of Phragmites australis (L.) rhizosphere bacteria from contaminated site for bioremediation of colored distillery effluent. Ecol. Eng. 27, 202-207.
- Clarke, T.R., Owens, N.J.P., 1983. A simple and versatile micro-computer program for the determination of most probable number. J. Microbiol. Methods 1, 133-137.
- De Muynck, W., Debrouwer, D., De Belie, N., Verstraete, W., 2008a. Bacterial carbonate precipitation improves the durability of cementitious materials. Cement Concrete Res. 38, 1005-1014.
- De Muynck,W., Cox, K., De Belie, N., Verstraete,W., 2008b. Bacterial carbonate precipitation as an alternative surface treatment for concrete. Constr. Build. Mater. 22, 875-885.
- Dick, J., DeWindt, W., DeGraef, B., Saveyn, H., VanderMeeren, P., DeBelie, N., Verstraete,
- W., 2006. Bio-deposition of a calcium carbonate layer on degraded limestone by Bacillus species. Biodegradation 17, 357-367.
- Diercks, M., Sand,W., Bock, E., 1991.Microbial corrosion of concrete. Experientia 47, 514-516.
- FHWA-RD-01-156, September 2001. Corrosion cost and preventive strategies in the United States, Report by CC Technologies Laboratories, Inc. to Federal Highway Administration. (FHWA), Office of Infrastructure Research and Development.
- Gerilla, G.P., Teknomo, K., Hokao, K., 2007.An environmental assessment of wood and steel reinforced concrete housing construction. Build. Environ. 42, 2778-2784.
- Gollapudi, U.K., Knutson, C.L., Bang, S.S., Islam, M.R., 1995. A new method for controlling leaching through permeable channels. Chemosphere 30, 695-705.
- Gross, A., Kaplan, D., Baker, K., 2007. Removal of chemical and microbiological contaminants from domestic greywater using a recycled vertical flow bioreactor (RVFB). Ecol. Eng. 31, 107- 114.
- Jugnia, L.B., Cabral, A.R., Greer, C.W., 2008. Biothic methane oxidation within an instrumented experimental landfill cover. Ecol. Eng. 33, 102-109.
- Neville, A.M., 1996. Properties of Concrete. Pearson Higher Education, 4th edition. Prentice Hall, New Jersey.
- Ramachandran, S.K., Ramakrishnan, V., Bang, S.S., 2001. Remediation of concrete using micro-organisms. ACI Mater. J. 98, 3-9.
- Ramakrishnan, V., 2007. Performance characteristics of bacterial concrete-a smart biomaterial. In: Proceedings of the First International Conference on Recent
- Advances in Concrete Technology,Washington, DC, 2007, pp. 67-78.
- Rodriguez-Navarro, C., Rodriguez-Gallego, M., BenChekroun, K., Gonzalez-Munoz, M.T., 2003. Conservation of ornamental stone by Myxococcus xanthus-induced carbonate biomineralization. Appl. Environ. Microb. 69, 2182-2193.
- Schlegel, H.G., 1993. General Microbiology, 7th edition. University Press, Cambridge.
- Soltmann, U., Raff, J., Selenska-Pobell, S., Matys, S., Pompe, W., Boettcher, H., 2003.
- Biosorption of heavy metals by sol-gel immobilized Bacillus sphaericus cells, spores and S-layers. J. Sol-Gel Sci. Technol. 26, 1209-1212.
- Stocks-Fischer, S., Galinat, J.K., Bang, S.S., 1999. Microbiological precipitation of CaCO3. Soil Biol. Biochem. 31, 1563-1571.
- Worrell, E., Price, L., Martin, N., Hendriks, C., Ozawa Meida, L., 2001. Carbon dioxide emissions from the global cement industry. Annu. Rev. Energy Environ. 26, 303-329.
Authors
Henk M. Jonkers(a), Arjan Thijssen(a), Gerard Muyzer(b), Oguzhan Copuroglu(a), Erik Schlangen(a)
a - Delft University of Technology, Faculty of Civil Engineering & Geosciences - Department of Materials & Environment, Stevinweg 1, 2628 CN Delft, The Netherlands
b-Department of Biotechnology, Faculty of Applied Sciences, Julianalaan 67, 2628 BC Delft, The Netherlands