Steel-Plate Composite (SC) Construction
Steel-plate composite (SC) construction, which requires prefabricated steelplate type structural modules, provides obvious benefits of modular construction leading to schedule contraction.
Introduction
The March 2011 events at Fukushima nuclear power plant have underscored the importance of resiliency of nuclear power plants for beyond-design-basis events (especially seismic related). As a consequence, the resiliency of existing fleet of U.S. plants for various types of external hazards is being (or will be) investigated using Probabilistic Risk Analysis (PRA) evaluation or other suitable techniques. In case the plant's existing design basis for those hazards is deemed inadequate, then appropriate plant upgrades, if needed, will be identified and implemented to reduce the plant's risk profile.
For new nuclear power plants, there is greater expectation of safety and resiliency (e.g., impact loading due to a commercial jetliner), with stipulation of withstanding a range of man-made and natural disaster impacts. Similarly, there is an increasing expectation that the design and construction of the plants incorporate more sustainability features, including minimizing the carbon footprint and material use.
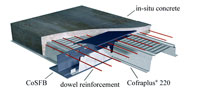 |
There are some options for achieving these goals to a varying degree: (i) improve resiliency of the traditional reinforced concrete (RC) design by using thicker walls and/or additional rebar and increase sustainability using "green concrete", |
(ii) improve resiliency by using seismic isolation methods to reduce the seismic demands on structures and supported commodities, or (iii) use steel-plate composite (SC) construction to address both resiliency and sustainability aspects. While RC structures are often the default option, the SC structures option has been gaining attention in recent years because it provides modular construction and schedule contraction advantages while also leading to a robust design against seismic, blast, and impact loads. Several Standard Plant suppliers for Advanced Light Water Reactor (ALWR) and Small Modular Reactor (SMR) vendors are either considering or have already incorporated the use of SC members (primarily SC walls) in their designs. The seismic isolation option is also being explored by a few standard plant vendors, as it can provide a dramatic reduction in plant's seismic risk, and has the potential for seismic isolation of individual equipment (instead of plant/structure wide seismic isolation).
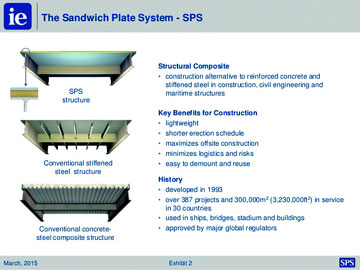
|
The SC Option – A Different Approach for Nuclear Power Plant Construction
There is renewed interest in nuclear power in the U.S. and the world because of growing concerns of global warming and energy security. Significant level of new nuclear power plant construction has been ongoing for the past several years, and the trend is expected to grow further. For this trend to be maintained, resiliency, sustainability, and affordability issues need to be addressed because construction could be underway for multiple numbers of projects. With this in mind, the nuclear power industry is exploring new and innovative approaches to optimize the constructability of new power plants. It appears likely that the use of modular mechanical and structural components will provide the single most significant area of cost and schedule savings for the next generation of power plants.
Figure 1: Illustration of RC and SC Construction for walls (from MPR-2610, a report to DOE)
http://www.power-eng.com/content/dam/NPI/Print%20Articles/2015/04/npi1.jpg
Steel-plate composite (SC) construction, which requires prefabricated steel-plate type structural modules, provides obvious benefits of modular construction leading to schedule contraction. As illustrated in Figure 1, the steel faceplates serve as formwork during concrete placement and, and through composite action enabled by shear connectors they also serve as equivalent reinforcing steel (rebar) during the service life of the structure. The use of faceplates thus eliminates formwork and rebar while facilitating fabrication of large empty modules that can be brought to their final location in the field for expeditious concrete placement (see Figure 2). Such modular composite construction approach has already been incorporated by some standard plant suppliers, and now with the impending issuance of the first (AISC-issued) US consensus standard for its design, SC construction usage is likely to expand further. The schedule advantage of SC construction is well understood, and is the prime reason for its expanding embrace in the nuclear industry. The use of large scale and well planned/executed SC construction offers a promising opportunity for also delivering increased resiliency and sustainability benefits.
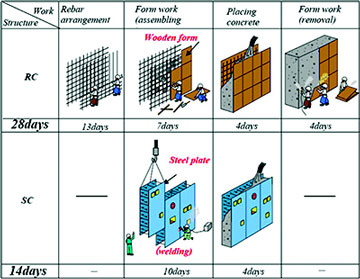 |
Figure 2: Illustration of Construction Activities for RC Wall and SC Wall (from MPR-2610, a report to DOE)
http://www.power-eng.com/content/dam/NPI/Print%20Articles/2015/04/npi2.jpg
Background
Nuclear power plant construction involves extensive use of reinforced concrete (primarily because it is a cheap option for radiation shielding). As a result, the construction schedule has been traditionally prolonged due to extensive and complex rebar cage fabrication, erection, and disassembly of elaborate concrete formwork. A 2004 report by the U.S. Department of Energy ("Study of Construction Technologies and Schedules, O&M Staffing and Cost, Decommissioning Costs and Funding Requirements for Advanced Reactor Designs") conservatively estimates that use of SC modules in place of conventional reinforced concrete can reduce total construction time by at least several months. SC modules typically have steel on the outside to act as stay-in-place formwork and equivalent rebar, thus eliminating the associated labor and schedule-intensive activities. Extensive use of prefabrication and modular construction in Asian power plants has already significantly expedited construction times, reduced overall costs, and optimized energy and material use. In Japan, use of modular techniques has demonstrated that the total construction time can be reduced to about 4 years for new plants (see 2001 DOE report entitled "A Roadmap to Deploy New Nuclear Power Plants in the United States by 2010, Volume II"), compared to a typical duration of 5 to 6 years. Four-year construction duration is also the target for the upcoming new generation of nuclear plants in the U.S.
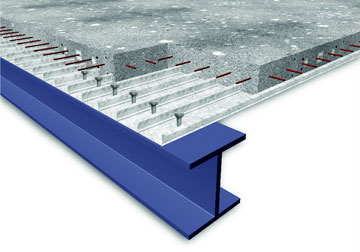
|
SC modules typically have steel on the outside to act as stayinplace formwork and equivalent rebar, thus eliminating the associated labor and schedule-intensive activities. |
Westinghouse Electric Corporation (WEC) has incorporated SC modules for walls and floors of its AP1000 plant internal structures, and was the first to incorporate them in a certified design (see WEC's design certification document, DCD, for AP1000 standard plant). A typical wall module panel for the AP1000 plant containment internal structures is shown in Figure 3. Figure 4 shows a pre-assembled internal structure SC module. WEC also extended the use of SC modules to the AP1000 shield building to make it resilient against aircraft impact, which is a stipulated beyond-design-basis (BDB) event for new nuclear power plants.
Figure 3: Typical Structural Wall Module in AP1000 Plant (from AP1000 DCD)
http://www.power-eng.com/content/dam/NPI/Print%20Articles/2015/04/npi3.jpg
Construction of several AP1000 units is currently underway in the US and China. Korea Hydro and Nuclear Power (KHNP) is incorporating some SC construction in its APR+ standard plant. MHI is doing the same for its APWR standard plant. Among SMRs, mPower and NuScale are currently exploring use of SC construction. SC design standards are in place in Japan and South Korea. The Europeans have recently begun developing their own SC research and codification program. The US standard will be issued by American Institute of Steel Construction (AISC) in September 2015. This development is expected to provide additional impetus for increased usage of SC construction in nuclear power plants. ASCE nuclear standards committee is in the process of endorsing the use of SC structures for DOE nuclear facilities, which is expected to further expand the SC usage.
Outside of nuclear facilities, SC walls are being used in commercial buildings as wall piers for seismic resistance (and ease of construction). In the UK, they are also being used for defense/secured facilities to improve structures' blast and impact resistance, an application that is being considered in the US as well.
General Benefits and Unique Considerations for SC Construction
There are many obvious benefits of SC construction related to cost and schedule reduction. The following advantages are generic to all types of modular SC construction:
- Steel faceplates, acting as forms, enable prefabrication of large, ready-for-placement modules.
- Elimination of rebar and formwork minimizes field labor and reduces schedule duration.
- Increased shielding capability enables up to 10 percent reduction in wall thickness, reducing some concrete quantity.
- Quality of placed concrete is generally superior, as there are no problems associated with rebar congestion and there are no issues associated with moisture loss due to evaporation.
- Concrete presence between steel plates ensures very good fire resistance.
- Penetrations can be handled easily during initial construction; minor penetrations can be handled relatively easily during service life.
- Major attachments (e.g., for large bore pipe supports) can be easily incorporated during initial construction.
- Minor/moderate attachments to faceplates can be easily handled during service life (e.g., for small pipe and conduit supports) since the faceplates serve similar function as embed plates (unlike SC construction, they have to be specifically provisioned in RC construction).
- High in-plane membrane (axial and shear) strength and ductility are achieved.
- Improved resistance to out-of-plane (bending and shear) loads.
- No curing is needed for SC wall construction since the faceplates prevent loss of moisture.
- Faceplates provide leaktightness, which protects concrete during service life (liner plates are already needed for applications where a wall is in contact with water on one or both faces; SC walls leverage these liner plates by making them structural steel reinforcement and leakage barriers for the walls).
- There are several considerations that are unique to SC construction. They are discussed below.
- Degree of Modularization and Module Fabrication Process: The full promise of SC construction is better realized if large modules are used. Prefabrication of large modules is ideally accomplished at a shipyard facility in preparation for module delivery by barge to the construction site. Even if access to existing shipyards is available, delivery by barge can difficult because many candidate sites do not have barge access. In such cases, an onsite fabrication shop could be set up to further assemble truckable SC panels into larger modules.
- Module Design for Construction Loads: It is important that the modules be designed for anticipated handling and rigging loads. Empty modules have lower strength and stiffness; therefore, adequate strength and stiffness need to be designed into the system by considering/stipulating the anticipated handling/rigging loads. Since large modules are very heavy and have complex geometries, large crane capacity and specialized rigging supervision is needed at the site to ensure safe handling.
- Concrete Placement: Free-fall placement method works well for placing normal concrete between the faceplates. However, self-consolidating concrete (SCC) may need to be used for concrete placement in congested areas, such as in wall-to-floor and wall-to-basemat joint.
- The rate of concrete placement, which affects the magnitude of wet concrete pressure, is another parameter that must be controlled (as in any other formwork design). Typically, the steel faceplates are designed for 10 psi pressure when acting as forms. Excessive pressure not only causes high stresses in the plates, but can also lead to excessive permanent deformation (bulging) that can be undesirable from both aesthetic and functional standpoints (e.g., for making baseplate attachments). A pour rate of 6 to 8 feet per hour will typically ensure that a 10 psi pressure limit will not be exceeded unless the concrete is a very slow-setting type and/or placement temperatures are low. Increased pour rate and/or design for slower setting concrete mixes (e.g., due to cement substitutes such as fly ash) can be accommodated by having closer spacing of tie bars in the modules.
- Concrete drop height is another consideration. The quickest method of concrete placement for modular wall construction is use of a simple drop technique that also ensures good consolidation. A drop height of 10 to 20 feet is recommended. Specially located pour ports are needed if the module height exceeds 20 feet. Smaller aggregate size, made from hard rock, is preferable to ensure good placement using the drop technique. Specialized placement techniques may be used in potentially congested areas, such as wall-to-floor and wall-to-basemat joint locations.
- Fabrication/Welding/Field Fit-up: Modules are formed by splicing adjoining SC panels (made from plates that may be about 8-10 ft wide and 40-60 ft long). Module fabrication is generally accomplished in a shop. Various modules are then installed in the field and connected with each other (or with other structural elements such as basemat) using special connection methods. The field erection and connection require dealing with fit-up and tolerances (similar to tank and vessel construction). The fit-up issues can be minimized using good welding practices (even minimizing welding where possible) and through smarter design of connections.
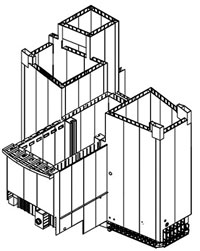
|
Figure 4: Example of Large Pre-Assembled Module in AP1000 Plant (from AP1000 DCD)
http://www.power-eng.com/content/dam/NPI/Print%20Articles/2015/04/npi4.jpg
Improved Sustainability and Resiliency
SC construction has certain natural benefits related to resiliency and sustainability. As discussed below, some of these benefits can be further realized with additional design features and construction planning.
The following considerations apply for improved resiliency of SC structures:
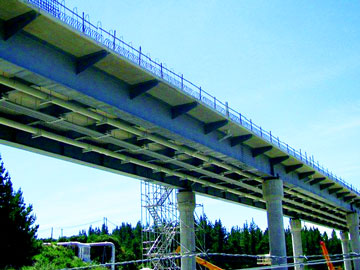
|
SC construction generally results in increased strength and ductility (for comparable amount of rebar/steel density per unit volume of concrete). |
- Resiliency for Missile and Blast Loads: The presence of faceplates naturally leads to increased missile and blast resistance. These attributes can be enhanced with better design of faceplate tying system in terms of strength, spacing, and ease of fabrication of tie-bars.
- Resiliency for External Hazard Loads: SC construction generally results in increased strength and ductility (for comparable amount of rebar/steel density per unit volume of concrete). This naturally leads to higher margins against design basis external hazard loads (e.g., seismic, high wind). This benefit can be best realized if full strength connections are used to join SC elements with other connected members (e.g., basemat) and if the steel ratio (i.e., combined thickness of steel faceplates divided by the total thickness of SC wall) is kept between 2% to 4%.
- Resiliency for Accident Thermal Loads: The accident thermal load, resulting from a loss-of-coolant or mains steam break event, leads to exposure to elevated temperature for sustained duration. This loading poses some more challenge to SC members since the faceplate is immediately exposed to the elevated temperature (i.e., there is no concrete cover). A seismic event could occur during such extended exposure (which is a BDB consideration). Performance against these scenarios can be improved by using full strength connections, steel or fiber reinforced concrete, or fire-resistant steel plates.
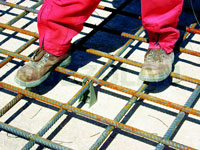 |
Durability and Long Service Life: The faceplates provide leaktight protection for concrete. However, just like any steel structure, the exposed steel surfaces need to be protected from corrosion. The protection scheme will depend on the service environment (e.g., interior v/s exterior walls and underground walls). |
- Resiliency against Exposure to Fire: The faceplate that gets exposed to fire loses strength and stiffness; however, similar to RC construction, the bulk of concrete and the opposite faceplate maintain good strength and stiffness. As such, SC structures have better fire resistance than pure steel structures; however, the fire resistance can be enhanced if suitable fire protection coating is applied to the faceplate(s) or fire-resistant steel is used. The member's strength and stiffness during fire exposure can also be improved by embedding another steel plate within the concrete (i.e., between the faceplates).
The following considerations apply for improved sustainability of SC structures:
- Field Labor Force Reduction: SC construction requires smaller field labor force compared to conventional SC construction because of elimination of activities related to formwork, rebar election, and curing. As in any modular construction, the degree of field labor reduction depends on the extent of modularization and the size of modules. Use of automated welding techniques, field bolting can help further in this regard. With maximization of field labor reduction, there is a commensurate reduction in the carbon footprint associated with mobilization, demobilization, and daily life of field labor force, etc.
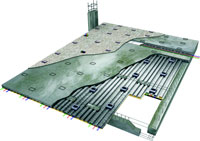 |
Reduction of Water Usage: SC construction does not have rebar congestion issues such that the amount of water used in concrete mix design can be reduced without sacrificing placement quality issues. Also, because no curing is required, the associated water usage is eliminated. |
- Use of Green Concrete or Cement Substitutes: Cement is an energy-intensive ingredient of concrete. Green concrete mixes, involving replacement of cementitious materials or naturally occurring cement substitutes (e.g. fly ash, pozzolans, slag) is always desirable from sustainability standpoint. The slow setting pace of cement substitutes can be easily handled in SC design by ensuring that the faceplates are adequately designed as formwork (the slow setting pace can be problematic in conventional RC construction in that the form stripping and subsequent placement activities have to be delayed until the concrete gains sufficient strength).
- Prolonged Service Life: SC structures will have long service life provided that potential for steel plate corrosion is addressed. Depending on the application (e.g., underground wall or just exterior wall exposed to atmosphere or walls exposed to water), certain types of stainless steel can be used advantageously. Compared to surface treatment options (e.g., cathodic protection or surface coatings), the stainless option may be most cost efficient in the long run.
- Surface Decontamination: The ability to decontaminate the exposed surfaces and the effort required to do so is an important consideration in any nuclear facility. The presence of faceplates lends for easy decontamination process during service life and after decommissioning. Faceplate surface treatment and material selection can be especially based on ease of decontamination. This is a sustainability consideration in terms of controlling potential spread of radioactive materials.
- Potential for Recycling: Unlike rebar in RC construction, the steel faceplates can be more easily removed and recycled. Also, the concrete can be pulverized and used in rubblized pavement construction.
- Reduced Energy Use during Construction: SC construction has reduced need for concrete compaction (consolidation using vibrators) since the free fall placement method generally leads to well-consolidated concrete. Also options for field bolting can be maximized to reduce energy consumption during construction.
- Material Savings in Steel and Concrete Quantity: Owing to their higher strength and radiation shield capability, SC member design can be optimized to produce reduced amount of steel and concrete compared to comparable RC member. This aspect has to be exploited during the design phase (which is often not done in SC design). The quantity reductions have obvious sustainability implications.
- Elimination of Water-Stops and Rebar Accessories: Unlike conventional RC construction, SC construction has no need for water-stops (because of its inherent leaktightness) and accessories such as rebar chairs. This results in reduction of the rubber and plastic based materials, which is good in terms of sustainability.
- Increased Potential for Modular Attachment of Supported Commodities: The SC modules can be designed to further enhance the ability to support/attach piping/equipment modules, structural modules (e.g., for floors/platforms). This can lead to further reduction in the associated labor forces and energy use in the field (aside from the resulting schedule contraction).
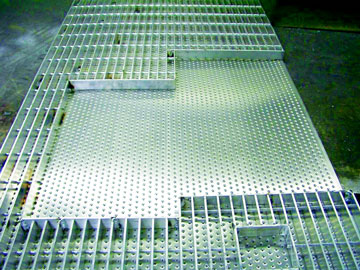 |
- Use/Reinvigoration of Nation's Shipyard and Fabrication Facilities: Fabrication of SC modules could be performed at a shipyard facility or at a steel fabricator shop experienced in nuclear related steel fabrication. This will be a boost for idled or under-utilized fabrication facilities, and will provide new job opportunities.
Conclusion
Resiliency and sustainability are highly desired features for future nuclear power plant structures. There are a few construction options to incorporate these considerations. The SC construction option provides certain inherent benefits related to schedule and material savings, and provides strong promise for many attributes related to resiliency and sustainability. The resiliency and sustainability benefits of SC construction can be maximized by implementing a well thought out design and construction approach.
Authors
Sanjeev R. Malushte, Bechtel Fellow and Technology Manager, Bechtel Corporation
Amit H. Varma, Professor of Civil Engineering, Purdue University
Acknowledgement
This article has appeared before in www.power-eng.com dated 08/25/2015. Reproduced here for reader’s benefit.